On this page: The Rocket > Liftoff to Spaceflight > In Orbit > Free falling > Interplanetary Trajectories
From Newton's Cannon to Spaceflight
Our knowledge of orbital mechanics helped us to understand what is necessary to keep satellites in place near the Earth, enabled humans to travel to the Moon, and send out probes to every planet in the solar system. The human path to space was indeed a long one, today space accomplishments seem to come a lot easier. So far, a method of placing satellites in orbit that doesn't involve launching spacecraft from the Earth's surface into orbit does not exist.
Navigate Astra's Guide to Orbits
Introduction > Kepler's Laws of Planetary Motion > Newton's Laws of Motion and Gravity > Spaceflight > Identifying Orbits > Lagrange Points > Orbital Elements > Orbit Terminology > Orbit Info and Resources
The Rocket Lifts Us Above the Earth
In order to escape Earth's surface, a rocket provides the means to accelerate a spacecraft. In order to achieve flight, a rocket uses a fuel, engines, a combustion chamber, and a nozzle that creates thrust by expelling mass to take advantage of Sir Isaac Newton's third law. Combustion increases the temperature of gas in the engine, whereupon it expands and rushes out through the nozzle. The vehicle is accelerated in the opposite direction. There are basically two kinds of rockets, some use liquid fuels but others use a solid rocket fuel.
The rocket anatomy image shows the basic parts of a rocket. Astra's rocket is simplified to show the basic rocket design plan. Most modern rockets have complex engines using pumps that help increase the pressure of the fuel in the combustion chamber, resulting in more thrust. Modern rockets don't use large fins as shown here, they maintain stability by swiveling or "gimboling" the nozzle(s).
To reach orbit, the rocket must lift its payload up to 17,500 miles per hour or 7.82 km per second. The rocket is able to move faster as it raises higher because the spent propellent has less mass and the Earth's atmosphere becomes thinner as it rises. In general, a spacecraft is said to reach space when it rises 62 miles above the Earth's surface or 100 km.
When a rocket is standing at the launchpad, its height above the surface before it lifts off is its perigee. If the rocket has enough fuel to reach orbit it will miss Earth's surface at perigee by a greater margin when it returns to the place it lifted off. Launching from the Earth's surface means the velocity of its orbit is added to the spacecraft's speed. That's the general idea of how a rocket gets to space, but what does a spacecraft or satellite have to do when it gets up there?
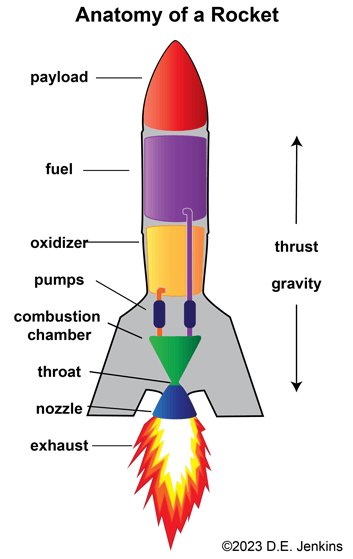
From Liftoff to Orbital Spaceflight
Orbital motion is obtained by giving an object enough horizontal velocity so that by the time gravity pulls it down, it has traveled far enough that Earth’s surface curves away from it. It stays above the surface. An object in orbit is falling around the Earth but going so fast that it never hits it.
A rocket can get spacecraft into orbit, using one or more boosters. When a booster's job is done, it is left behind. In the past, the spent boosters were just left to burn up in the Earth's atmosphere. The Space Shuttle dropped its solid rocket booster to be retrieved from the ocean, but SpaceX developed the Falcon 9 rocket that has been launched and returned to Earth more than 200 times. (Some of the individual Falcon boosters have been returned to Earth over 15 times!)
A spacecraft can change its energy by firing its thrusters. It thrusters are fired parallel to the direction of travel (the thrusters point behind it) it will increase velocity. Point the thrusters against the direction of travel (with the thrusters in front of it) and it will slow down. Changing the orbit is called "Delta V" (indicated by using a Greek Delta symbol, "Δ".)
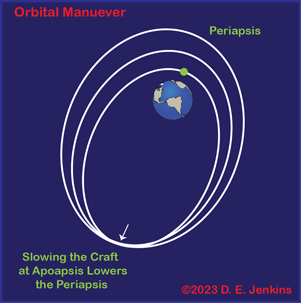
When the spacecraft is in orbit following an elliptical path, the spacecraft's periapsis altitude can be raised by increasing the spacecraft's energy at apoapsis. This is done by firing smaller on-board rocket thrusters when at apoapsis. Applying more energy at apoapsis will raise the periapsis altitude. Increasing speed at periapsis will also boost the spacecraft's altitude at apoapsis.
If the spacecraft fires thrusters and decreases its energy at apoapsis, that will lower the periapsis altitude just as applying more energy at periapsis, the spacecraft can raise its apoapsis altitude. Studying the diagram reveals that the spacecraft stays where it is at apoapsis when the thrust was applied. It is getting closer to the surface of the Earth based on its perigee.
The opposite is also true, by decreasing energy at periapsis, the spacecraft can lower the apoapsis altitude. If Newton's cannonball skimmed through the tops of some trees as it passed through periapsis, the slowing effect would rob energy from the cannonball, and it could not continue to climb to quite as high at apoapsis as it did before.
In practice, by removing energy from a spacecraft's orbit at periapsis by using the onboard rocket thrusters at that point use up propellant. By intentionally and carefully dipping into a planet's atmosphere, frictional drag can slow down the spacecraft. This is called aerobraking, a technique used at Venus and at Mars that conserves rocket propellant. It helps spacecraft travel long distances using less fuel.
During NASA's Artemis-1 mission, the Orion capsule used a "skip entry" a special aerobraking maneuver. On returning to Earth, it dipped into the upper part of Earth’s atmosphere. It used that atmosphere and the lift of the capsule, to skip back out of the atmosphere, then reenter for final descent. This manuever was a bit like skipping a rock across the water in a river or lake, and enabled the capsule to travel closer to the United States using less propellent.
The key to spaceflight is to make changes in velocity by applying energy to the spacecraft when it is at the important positions of apoapsis and periapsis in its orbit. There are other important positions where changes can be made to a spacecraft's orbit, we'll look at these later in Astra's Guide to Orbits.
Orbiting a Planet
Isaac Newton's cannonball is a good analogy as it makes it clear that to get a spacecraft into orbit, it must raise up and accelerate until it is going so fast that when it falls, it falls completely around the Earth. Rockets normally launch from a vertical position, rising from the launch pad straight up then quickly roll to take a curved trajectory to orbit. It is a maneuver known as a "gravity turn." A gravity turn, also known as a zero lift-turn, is a maneuver that uses the Earth’s gravity during the launch to put the rocket on the correct trajectory to establish the desired orbit around the planet. It can save propellent and time because the slight roll and tilt move the rocket in a specific direction.
In practical terms, the spacecraft can be less than about 150 kilometers above the surface of Earth. At that altitude, the atmosphere is so thin that it doesn't present much frictional drag to slow the spacecraft down. The rocket must accelerate to speed the spacecraft up to the neighborhood of 30,000 km/hr or about 19,000 mph. At that speed, the spacecraft will continue to fall around Earth. No more propulsion is necessary, except for occasional minor adjustments. It can remain in orbit for months or years before the presence of the thin upper atmosphere causes the orbit to degrade. These same mechanical concepts (but different numbers for altitude and speed) apply whether orbiting Earth, Venus, Mars, the Moon, the sun, or any other object.
Other Names for Apsides
As mentioned in the discussion of apsides in the Astra's Orbit Guide, periapsis and apoapsis are generic terms. The prefixes "peri-" and "ap-" are added to the Greek or Roman names of the bodies that are being orbited. For example, we use perigee and apogee for orbiting Earth, perijove and apojove at Jupiter, periselene and apselene or perilune and apolune in lunar orbit, pericrone and apocrone if you're orbiting Saturn, and perihelion and aphelion if you're orbiting the Sun. For binary stars in orbit with each other, the term apastron and periastron are used. Undoubtedly, there are more ways to discuss the concept out there in the universe.
Freefall
An object that falls through a vacuum is subjected to only one force - - the gravitational force. An object that is moving only because of gravity is said to be "free falling" and its motion is described by Newton’s second law of motion. The acceleration of a free falling object is always equal to the acceleration due to gravity, 9.81 m/s2. Free fall is not affected by the weight, size, or shape of the object.
One can experience the sensation of freefall by riding a roller coaster as it is going over a tall hill and falling on the other side. During the time from right before the coaster goes over the hill and falls down the other side, coaster riders are in freefall. A person who is sky diving is in free fall until they open the parachute. Astronauts in orbiting spacecraft are in free fall. They are falling at the same rate as the spacecraft. Untethered objects appear to be floating (falling) beside them or around them if they are aboard the International Space Station or another spacecraft.
A spacecraft in orbit has not escaped Earth's gravity. It is gravity that gives the mass the centripetal acceleration it needs to stay in orbit. Gravity is present and it is balanced out by the speed that the rocket provided when it placed the spacecraft in orbit. The force of gravity is still 90-95% in a vehicle in orbit. So while terms like "weightless" and "microgravity" are used, gravity is still dominant, but some of its familiar effects are not apparent in orbit.
Interplanetary Trajectories
To travel to distant planets, it's important to minimize the propellant mass needed by a spacecraft and its launch vehicle. This how interplanetary flight is possible using current launch capabilities by keeping the costs manageable. The amount of propellant needed depends largely on what route is chosen. Trajectories that by their nature need a minimum of propellant are therefore of great interest.
For a spacecraft to achieve Earth orbit, it must be launched to an elevation above the Earth's atmosphere and accelerated to orbital velocity. The most energy efficient orbit, that requires the least amount of propellant, is a direct low inclination orbit. To achieve this orbit, a spacecraft is launched in an eastward direction from a site near the Earth's equator. This takes advantage of the rotational speed of the Earth, it contributes to the spacecraft's final orbital speed. At the United States' launch site in Cape Canaveral, Florida (28.5 degrees north latitude), a due east launch results in a "free ride" of 1,471 km/h (914 mph).
Launching a spacecraft in a direction other than east or from a site far from the equator, results in an orbit of higher inclination. High inclination orbits are less able to take advantage of the initial speed provided by the Earth's rotation so the launch vehicle must provide a greater part, perhaps all, of the energy required to attain orbital velocity. Although high inclination orbits are less energy efficient, they must be used instead of equatorial orbits for some applications.
Author's note, some of the information presented here can be found on Basics of Spaceflight from the NASA Jet Propulsion Laboratory website and Newton's Principles of Mechanics from the NASA Solar System Exploration website. Of course, the content has been adapted here for Astra's Stargate. For more details, use the Orbit Information and Resources page.
#AstrasStargate #ImaRocketFan
This webpage is ©2023 D. E. Jenkins all rights reserved. Please use the contact page to get permission to use this content or to send comments or corrections.
Navigate Astra's Guide to Orbits - or return to Orbits home